In this page we give an introduction on the CASCADE model main goals and framework. The informations supplied are designed to just give a basic idea on the model functioning, and are not exaustive. We suggest to use the provided references and the CASCADE user manual to learn more.
CASCADE (CAtchment Sediment Connectivity And DElivery) is a flexible modelling framework which takes advantage of increasing availability of geomorphic data and hydrologic data in large-basins or at global scales to describe sediment connectivity in data rich as well as data scarce environments. (More on river sediment connectivity here…)
The CASCADE model is freely available to download with the CASCADE toolbox (Tangi et al., 2019). …More
Why using CASCADE?
Numerical models for sediment transport in rivers commonly require high-quality input data and large computational capacity. Such models are applicable to model sediment transport and hydrodynamics in well defined river segments and in “data rich” setting. Even where these data are available, considering single river reaches only does not take the connected nature of sediment transfers into account, i.e., from which possible remote sources sediment is delivered to a river segment.
In recent times, thanks to remote sensing technologies that supply consistent large-scale geomorphic data (Bizzi et al., 2018; Demarchi et al., 2017), numerical models of network-scale sediment connectivity emerged. (Betrie et al., 2011; Czuba, 2018; Czuba and Foufoula-Georgiou, 2015, 2014; Ranzi et al., 2012).
CASCADE (CAtchment Sediment Connectivity And DElivery) (Schmitt et al., 2016) has the ambition to represent some of the complexity of network scale sediment transfers while being parsimonious and applicable to large and data-scarce settings. E.g., CASCADE makes use of widely applied empirical formulas for describing the transport of many different grain sizes and scales their application to whole river networks. CASCADE also offers deterministic or stochastic approaches to model sediment inputs from the hillslope to river channels.
Previous applications included optimization of dam portfolios for minimal sediment trapping, identification of dominant sources of sediment for reservoir storage loss, and evaluation of basin-scale sediment connectivity alterations under different sediment management strategies.
CASCADE aims to match the increasing demand for large scale sediment transport assements for integrated river basin planning (Fryirs et al., 2007; Merritt et al., 2003) by building on numerical models of network-scale sediment connectivity (Czuba, 2018; Czuba and Foufoula-Georgiou, 2015, 2014). CASCADE also provides a framework for analyzing new types of multi-sclae sediment and geomorphic data that are available for entire basins from remote sensing technology (Bizzi et al., 2018; Demarchi et al., 2017).
How does it work?
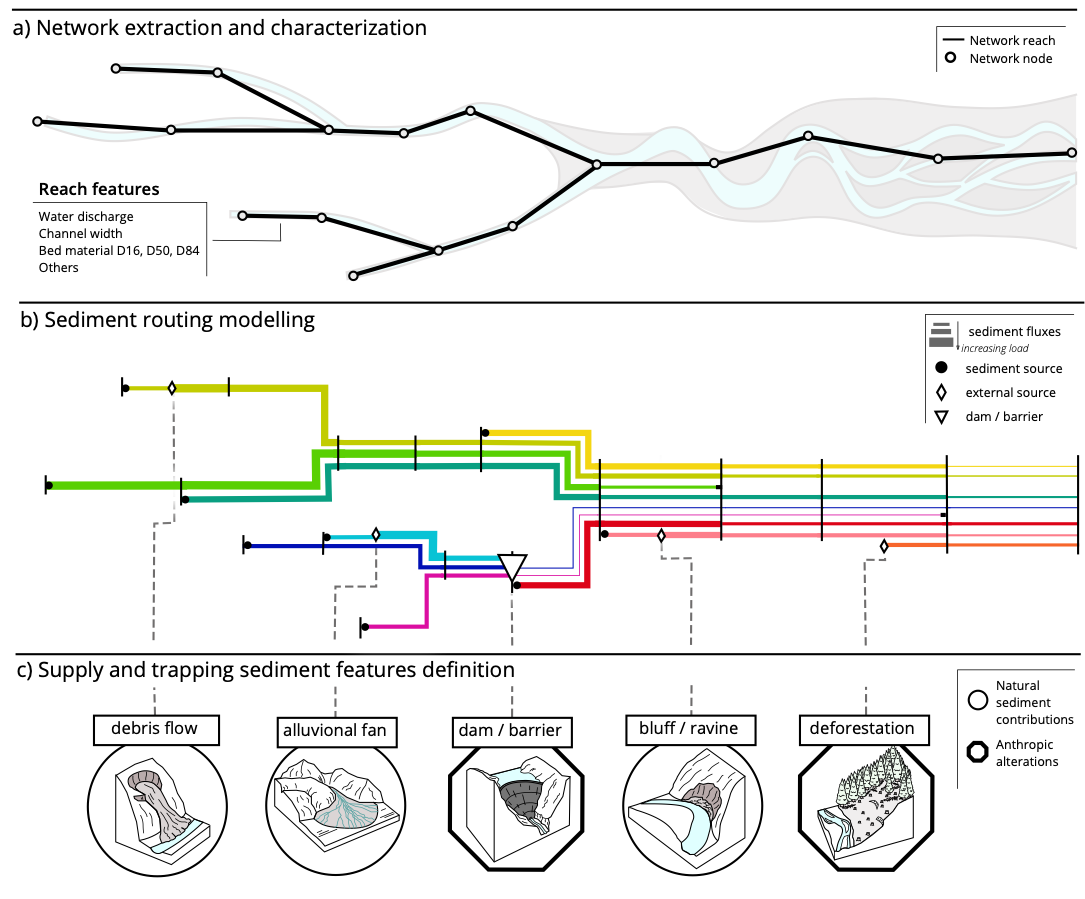
The figure above visualizes the basic steps employed by CASCADE to simulate sediment connectivity in a river network. As input, the model needs a representation of the river network (Fig a) as a directed graph consisting of nodes and reaches. In the network, each reach connects two nodes and represents a part of the river network with homogeneous geomorphic and hydraulic features. The network extraction is typically achieved from a DEM (Tarboton et al., 1991). Each reach is assigned a set of hydromorphologic attributes related to sediment transport.
Once the network is extracted and the reach features characterized, CASCADE can use these data to simulate sediment connectivity. CASCADE achieves this by describing sediment delivery through a combination of individual transport processes named “cascades ”. Each cascade derives from a source in the network (black dots in Fig b). Its path toward the outlet node of the network is defined by the network topology. A cascade transport a sediment flow and, while passing though the reaches, may lose part of its flow via deposition, according to the transport capacity of the reach it traverses (i.e. the amount of energy available in a reach for the transport of sediment of a specific size (Wilcock and Crowe, 2003; Yang et al., 2017), and the presence of other cascades, until either it depletes completely, or it reach the outlet.
The CASCADE model can consider for anthropogenic alterations in sediment connectivity (examples in Fig c). These are divided into two categories, 1) additional sediment supply to the network from the hillslopes or river banks (diamonds in Fig b) and 2) dams and barriers that retain sediment (triangle in Fig b). Additional sediment fluxes either generate a new cascade or increase the flow of the cascade originated in a reach. Dams are placed on a network node and cause the deposition of part of the load of the cascades traversing the barrier, according to their trap efficiency.
Need more info?
- Return to Learn More page
- Click here to download the CASCADE toolbox user guide
- Click here to access the literature about CASCADE
References
- Bizzi, S., Piégay, H., Demarchi, L., Van de Bund, W., Weissteiner, C. j., Gob, F., 2018. LiDAR-based fluvial remote sensing to assess 50–100-year human-driven channel changes at a regional level: The case of the Piedmont Region, Italy. Earth Surf. Process. Landf. 0. https://doi.org/10.1002/esp.4509
- Czuba, J.A., 2018. A Lagrangian framework for exploring complexities of mixed-size sediment transport in gravel-bedded river networks. Geomorphology 321, 146–152. https://doi.org/10.1016/j.geomorph.2018.08.031
- Czuba, J.A., Foufoula-Georgiou, E., 2015. Dynamic connectivity in a fluvial network for identifying hotspots of geomorphic change. Water Resour. Res. 51, 1401–1421. https://doi.org/10.1002/2014WR016139
- Czuba, J.A., Foufoula-Georgiou, E., 2014. A network-based framework for identifying potential synchronizations and amplifications of sediment delivery in river basins. Water Resour. Res. 50, 3826–3851. https://doi.org/10.1002/2013WR014227
- Demarchi, L., Bizzi, S., Piégay, H., 2017. Regional hydromorphological characterization with continuous and automated remote sensing analysis based on VHR imagery and low-resolution LiDAR data. Earth Surf. Process. Landf. 42, 531–551. https://doi.org/10.1002/esp.4092
- Kondolf, G.M., 1997. Hungry Water: Effects of Dams and Gravel Mining on River Channels. Environ. Manage. 21, 533–551. https://doi.org/10.1007/s002679900048
- Schmitt, R.J.P., 2016. CASCADE – A framework for modeling fluvial sediment connectivity and its application for designing low impact hydropower portfolios. Politecnico di Milano, Milan, Italy. https://www.politesi.polimi.it/handle/10589/131002
- Schmitt, R.J.P., Bizzi, S., Castelletti, A., 2016. Tracking multiple sediment cascades at the river network scale identifies controls and emerging patterns of sediment connectivity. Water Resour. Res. 52, 3941–3965. https://doi.org/10.1002/2015WR018097
- Tarboton, D.G., Bras, R.L., Rodriguez‐Iturbe, I., 1991. On the extraction of channel networks from digital elevation data. Hydrol. Process. 5, 81–100. https://doi.org/10.1002/hyp.3360050107
- Wilcock, P.R., Crowe, J.C., 2003. Surface-based Transport Model for Mixed-Size Sediment. J. Hydraul. Eng. 129, 120–128. https://doi.org/10.1061/(ASCE)0733-9429(2003)129:2(120)
- Yang, H., Lin, B., Sun, J., Huang, G., 2017. Simulating Laboratory Braided Rivers with Bed-Load Sediment Transport. Water 9, 686. https://doi.org/10.3390/w9090686
- Tangi, M. , R. J., Bizzi, S. & Castelletti, A. (2019). The CASCADE toolbox for analyzing river sediment connectivity and management. Environmental Modelling & Software. [DOI: 119. 10.1016/j.envsoft.2019.07.008.]